Tailored Nutrition The Science Behind Prescription Dog Diets
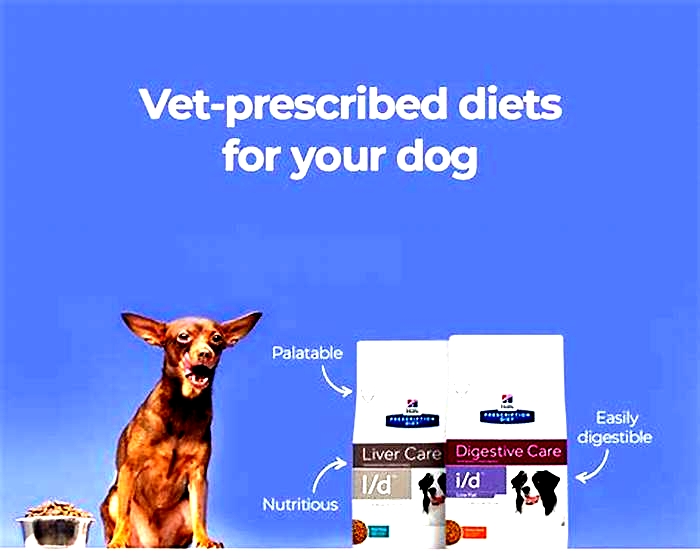
Genetic test for the prescription of diets in support of physical activity
Acta Biomed. 2020; 91(Suppl 13): e2020011.
Genetic test for the prescription of diets in support of physical activity
,1 ,2 ,3 ,3 ,3 ,3 ,4 ,4 and 4,5,6
Zakira Naureen
1 Department of Biological Sciences and Chemistry, College of Arts and Sciences, University of Nizwa, Nizwa, Oman
Giacinto Abele Donato Miggiano
2 Human Nutrition Research Center, Sacro Cuore Catholic University, Rome, Italy
Barbara Aquilanti
3 UOC Nutrizione Clinica, Fondazione Policlinico Universitario A. Gemelli IRCCS, Rome, Italy
Valeria Velluti
3 UOC Nutrizione Clinica, Fondazione Policlinico Universitario A. Gemelli IRCCS, Rome, Italy
Giuseppina Matera
3 UOC Nutrizione Clinica, Fondazione Policlinico Universitario A. Gemelli IRCCS, Rome, Italy
Lucilla Gagliardi
3 UOC Nutrizione Clinica, Fondazione Policlinico Universitario A. Gemelli IRCCS, Rome, Italy
Alessandra Zulian
4 MAGIS LAB, Rovereto (TN), Italy
Roberta Romanelli
4 MAGIS LAB, Rovereto (TN), Italy
Matteo Bertelli
4 MAGIS LAB, Rovereto (TN), Italy
5 MAGI EUREGIO, Bolzano, Italy
6 EBTNA-LAB, Rovereto (TN), Italy
1 Department of Biological Sciences and Chemistry, College of Arts and Sciences, University of Nizwa, Nizwa, Oman
2 Human Nutrition Research Center, Sacro Cuore Catholic University, Rome, Italy
3 UOC Nutrizione Clinica, Fondazione Policlinico Universitario A. Gemelli IRCCS, Rome, Italy
4 MAGIS LAB, Rovereto (TN), Italy
5 MAGI EUREGIO, Bolzano, Italy
6 EBTNA-LAB, Rovereto (TN), Italy
Received 2020 Sep 3; Accepted 2020 Oct 14.
Copyright: 2020 ACTA BIO MEDICA SOCIETY OF MEDICINE AND NATURAL SCIENCES OF PARMA
This work is licensed under a Creative Commons Attribution 4.0 International License
Abstract
Owing to the fields of nutrigenetics and nutrigenomics today we can think of devising approaches to optimize health, delay onset of diseases and reduce its severity according to our genetic blue print. However this requires a deep understanding of nutritional impact on expression of genes that may result in a specific phenotype. The extensive research and observational studies during last two decades reporting interactions between genes, diet and physical activity suggest a cross talk between various genetic and environmental factors and lifestyle interventions. Although considerable efforts have been made in unraveling the mechanisms of gene-diet interactions the scientific evidences behind developing commercial genetic tests for providing personalized nutrition recommendations are still scarce. In this scenario the current mini-review aims to provide useful insights into salient feature of nutrition based genetic research and its commercial application and the ethical issue and concerns related to its outcome. (www.actabiomedica.it)
Keywords: nutrigenetics, nutrigenomics, direct to consumer test, personalized nutrition, obesity, physical activity
Introduction
The notion that each of us has an exclusive nutrition blueprint within our genes is a scrumptious concept. Nutrients can indeed influence phenotypic expression of an individuals genotype by modifying gene expression at pre- and post-transcriptional and translational levels. This might result into improving health of an individual or, on the other hand, may affect the health in a negative manner. On the contrary there are certain genes that may give preferential benefit to intake of some of the nutrients while adversely affecting the consumptions of other. The complexity and girth of this scenario led to the development of two interesting scientific fields, nutrigenetics and nutrigenomics.
Nutrigenetics and nutrigenomics are defined as the branches of science dealing with the effect of genetic variation on nutritional response and the function of nutrients and bioactive food compounds in influencing gene expression, respectively (1-3). The wealth of genomic information coupled with high-throughput omics technologies facilitates the acquisition of in-depth knowledge of nutrient-gene interactions that ultimately lead to development of personalized nutrition approaches for good health and disease prevention (4-6). There are three fundamental factors underlying nutrigenetics and nutrigenomics. Genomic diversity with respect to ethnicity that affects nutrient bioavailability and metabolism, choice and availability of food depending on cultural, socioeconomical, geographical and sense of taste of an individual and malnutrition that can affect gene expression and pose threats to genome stability by paving the way for mutations in gene sequences or causing chromosomal aberrations resulting into abnormal gene dosage and corresponding adverse phenotypes (1-6).
Although the terms nutrigenomics and nutrigenetics look similar and are closely related, these terms are not interchangeable as nutrigenetics investigates the effect of inherited genetic traits or their variants and mutations on micronutrient uptake and metabolism while nutrigenomics is the interconnection between genome and diet which explains the nutritional effects on transcription, translation, proteomic, metabolomics changes and the variation in dietary factors due to individual genetic background (7, 8). Due to advancement in science now it is possible and affordable for individuals to get information about possibility of having alterations in genes involved in nutrient metabolism and pathways requiring micronutrients as cofactors, due to point or frameshift mutations or copy number variations (9). Age and gender may as well contribute to gene-diet interactions involved in micronutrient uptake for health maintenance (10). Hence the key challenge is to utilize this information to provide reliable and predictable personalized dietary recommendations for optimum health and wellbeing.
Another important emerging facet of gene-diet-interaction is involvement of epigenetic with the potential for both intra- and transgenerational effects (11,12). Epigenetics refers to regulatory mechanisms that play an important role in switching genes on and off thus having a strong impact on growth and development with epigenetic controls being lost during diseases such as cancer. Whereas epigenomics involves analysis of epigenetic changes in a cell or entire organism. Diet has a special perspective in this regard in the sense that either alone or in combination with confounding environmental factors it may switch certain genes on or off. Consequently , this may either lead to uncontrolled growth leading to cancer or predisposition to and susceptibility towards developing diseases later on in life (13,14). Thence the epigenome is the global epigenetic pattern that is heritable and modifiable by diet. The gene-specific modifications like DNA methylation, histone modifications and chromatin remodeling regulate the expression of house-keeping genes and suppress the expression of parasitic transposons (1,2,14).
This mini review aims to provides various perspectives on nutrigenomics and nutrigenetics and the use of technologies to unravel gene diet-interactions and their underlying mechanisms that result into inter-individual variations in response to the same nutritional intakes.
Genetic variation/polymorphisms affecting response to dietary intake
The term personalized nutrition is not a new concept. We have examples from inborn errors of metabolism that the nutrition regime can be customized for a healthy life. These inborn errors of metabolism are a consequence of genetic variations in the genes coding specific metabolic enzymes. Unavailability or non-functionality of these enzymes leads to metabolic problems. These variations can result in gene-diet-interactions and alter the nutrient requirements and the metabolism (4,5).
Classical examples of such single gene- diet- interactions affecting the response to dietary intake are phenylketonuria and lactose intolerance. Phenylketonuria is an autosomal recessive disorder caused by mutations in gene encoding a key hepatic enzyme phenyl alanine hydroxylase. Individuals having phenylketonuria need to avoid foods containing amino acid phenyl alanine as they lack or have very low activity of phenyl alanine hydroxylase to metabolize this amino acid (6). Another common example is the autosomal recessive lactose intolerance. This phenotype is caused by a point mutation in the lactase gene (LCT) that results into a T to C transition leading to the malfunctioning or absent lactase. Consequently, individuals that harbor this mutation are unable to digest lactose.
Besides these straight forward genetic interactions involving single genes and diet there are more complex polygenic interactions and multifactorial etiologies, such as obesity, cardiovascular disease (CVD), type 2 diabetes and cancer (1,2). Such disorders involve interactions among many genes and can be influenced by several dietary exposures. For instance multiple genetic variants have been linked with an increased risk of obesity such as fat mass and obesity associated gene (FTO, rs9939609), genes encoding peroxisome proliferator-activated receptor protein, uncoupling proteins (UCP1 and UCP3), leptin receptor and melanocortin 4 receptor (15-18).
In case of coronary artery disease variations in genes involved in lipid metabolism such as cholesteryl ester transfer protein (CETP); lipoprotein lipase (LPL), apolipoprotein E (APOE), low density lipoprotein receptor (LDLR) affect the uptake and catabolism of cholesterol and other lipids thus causing fat deposition in the arteries (atherosclerosis) that can be dangerous for health (19-22). Other genetic variants may be responsible for diabetes, cancer and other diseases (23-25). Another important example of metabolic pathways affected by genetic variants in genes encoding metabolic enzymes regards the variants that can be present in methyl tetrahydrofolate reductase gene (MTHFR). Two common variants in MTHFR C677T and A1298C have a minor allele frequency of 25% in Hispanic people and 10% in North American Caucasians. Both cause homocystenemia that eventually might result in birth defects, encephalopathies and glaucoma (26).
At present, there are many examples of how nutrigenomics is studying these complex disorders. One of those studies analyzed the involvement of genetic variants in the gene CYP1A2 encoding a caffeine-metabolizing enzyme in CVD (27). The study reported that high caffeine consumption may be associated with an increased risk of CVD in individuals carrying the slow caffeine metabolizing variant of the gene. On the contrary, individuals having fast coffee metabolizing genotype have a protective effect on moderate coffee consumption (27).
Substantial efforts have also been made in determining genetic variants in metabolic enzymes to devise effective optimal dietary regimes to prevent obesity, which may be a major contributory factor in chronic diseases such as CVD and type 2 diabetes. One example are the genetic variations in the apolipoprotein A2 gene (APOA2) that predisposes to obesity via altered energy intake (28). Individuals of Chinese and Asian-Indian origin with a specific variant in APOA2 are at increased risk of developing obesity when saturated fat intake is high, but not when saturated fat intake is low (28). Similar studies were replicated in a Mediterranean population from the southeast of Spain (29).
Additionally, polymorphisms in genes linked with the metabolism of vitamin C, D, B12 and iron, have been shown to predispose to risk of suboptimal or deficient blood levels of these nutrients leading to several abnormalities and diseases (30-33).
Despite the fact that these studies and many other similar studies provide strong evidence of benefits from personalised nutrition further research is required to deepen our knowledge of genetic association with dietary intake and physical activities in various ethnic groups (34,35). One of the ultimate objectives of nutrigenetics and nutrigenomics in this scenario is the development of genetic tests based on genetic markers. Research has shown that DNA-based personalised nutrition advice is taken more seriously and more willingly by the people in terms of alteration in behaviour and adapting to healthy lifestyle pattern (34,35).
Nutrigenetics-based personalized nutrition
Given the complexity of the matter, there is not a single definition for personalized nutrition, also called precision or tailored nutrition (36). Its aim is to give dietary advice in order to improve dietary habits (37). Personalized nutrition practices may involve direct face to face consultations or web based computer-generated nutrition advice (38). In this context, genetic-based personalized nutrition is a healthy tailored dietary recommendation based on genetic information collected by genetic testing (39). These data are coupled with lifestyle data such as age, gender, weight, height and clinical history for underlying diseases, food allergies, dietary habits and physical activity. Some centers also take into account gut microbiota (40). In fact, it can alter genetic expression in response to dietary intake and physical activity (40). Hence, personalized nutrition is a complex field (41).
Scientific validity and evidence for genotype-based dietary advice
In principle, to avoid misuse of the genetic information and protect the basic human rights of safety, privacy and well-being there should be clear guidelines to follow in nutrigenetics. Furthermore, these genetic findings should be translated carefully keeping in view the available scientific evidence. A draft framework for assessing the significance and practical application of the nutrigenetic knowledge was presented by Grimaldi et al (42). They proposed that based on this framework, transparent and scientifically valid guidelines might be developed for nutrigenetic testing for public.
They further added that genetic testing is mainly an unregulated market in the sense that they provide insufficient descriptions of results and draw inferences which lack of scientific backing (43-48). For instance, scientific studies addressing gene-diet interactions are mainly based on observations made for a group of individuals of a specific ethnic group or with mixed ethnicity. The results presented by a study are specific for that particular study group and cannot be directly applied to individuals with different ethnicity, unless these results are replicated in different ethnic groups with people having different life styles and dietary habits. This is due to the fact that the overall effect of confounding factors on an individual phenotype is far greater than a trend observed in a group of people. Besides that, diets prescribed on the basis of a single gene variant may also lack scientific backing. In fact, the overall contribution of a single gene in phenotypic expression is minimal as compared to multiple genetic variants and their interactions with each other (42). Therefore, the best personalized nutritional advice can be given only on the basis of a polygenic profile, phenotype characterization, health status, food choices, lifestyle, environmental, cultural and economic factors (43). To overcome these differences, dietary reference values have been developed to set safe limits for nutrients based on age, gender and physiological state (49-51). They are designed to meet the requirement of 97.5% of the population. This implicates that although these reference values cover the dietary requirements of most of the people some individuals may remain uncovered. In this context, EU developed a strategy to harmonize the nutrient recommendations throughout EU member states taking into account vulnerable groups and consumer satisfaction (52).
Interactions between diet and genetic variants
Wrong dietary habits such as high intake of sugars, fats, alcohol and reduced intake of vegetables and fruits may predispose to the acquisition of non-communicable diseases such as CVD, hypertension, diabetes, cancer and obesity (53).
Several epidemiological studies have consistently shown that high intake of sugar sweetened beverages (54-56), fried food (57) and sedentary life style are particular risk factor for obesity among adults and children due to their complex interaction with genetic variants associated with obesity (58-61).
Several genome wide association studies have assessed the association of genetic polymorphisms with metabolic pathways (62). For instance, clinically significant interactions have been observed among the APOA2 2265T>C variant and saturated fats intake and BMI (42); MTHFR gene variants and homocyteine levels (26,61-66); CYP1A2 genetic variants and hypertensive response to caffeine consumption.
Genome wide association and linkage studies have added more than 600 genetic variants and chromosomal regions to the repertoire of genetic factors associated with obesity, body weight, body mass index, body fat composition and distribution, energy expenditure and fuel oxidation and various other phenotypic features linked with nutrition (67-83). One of the first genetic variants associated with obesity was a single nucleotide polymorphism in the FTO gene. This variant affects body weight and body composition and carriers having FTO rs9939609 AA genotype are more likely to be obese as compared to non-carriers (71). Although this variant is the strongest risk factor associated with polygenic obesity, its effect can be modified by either physical activity or reduction in energy intake, providing an excellent example of cross talk between genetic variants and life style interventions (84). In addition to that another study revealed that patients carrying variant alleles of the FTO rs9939609 and MC4R rs17782313 are at a higher risk of type 2 diabetes mellitus (73). This implicates that the genetic variants can either affect the phenotype alone or in association with other genetic variants present in other loci or by complex interactions between genetic variants and environmental factors.
Genes and their polymorphs associated with obesity
One of the best method to achieve healthy weight loss is to create a calorie deficit by burning more calories than what we intake through diet. This method involves extensive and vigorous exercise or physical activity. On the other hand, intake of low calories with a more satiating diet may also result in weight loss in association with physical activity (67,81,83). Several genes have been reported to be linked with weight loss in response to hypocaloric diet coupled with physical activity. These genes mainly encodes important enzymes regulating lipid metabolism, adipogenesis, carbohydrate metabolism, circadian clock, energy intake and expenditure, appetite control, thermogenesis and cell differentiation (67-76). Furthermore, genetic variants in taste-, olfactory- and texture-related genes can influence sensitivity and preferences for certain food, thereby affecting the susceptibility towards nutrition-induced obesity (71). The key genetic variants that influence metabolic processes involved in increasing the risk of obesity and obesity-related diseases are FTO, ADIPOQ, LEP, LEPR, INSIG2, MC4R, PCSK1, PPARG, ADBR2, ADBR3, GHRL, PPAR, FABP2, APOA5, APOA1, LIPC, CETP, MTNR1B, NPY, GIPR, IRS1, TCF7L2, PCSK1 (84-120). The encoded proteins and their metabolic functions are presented in .
Table1.
Genes and their respective polymorphisms related to obesity and fat metabolism
Gene (OMIM ID) | Full name | SNP ID | Association | Reference |
FTO | Alpha-ketoglutarate dependent dioxygenase | rs9939609rs1558902rs8050136 | Stimulation of food consumption | 84-89 |
ADIPOQ | Adipocyte-, C1q-, and collagen domain-containing | rs1501299 | Energy expenditure | 90 |
LEP | Leptin | 164160 | Appetite regulation | 91 |
LEPR | Leptin receptor | rs1805094 | 92 | |
INSIG2 | Insulin-induced gene 2 | rs7566605 | Regulation of cholesterol and fatty acid synthesis | 93,94 |
MC4R | Melanocortin 4 receptor | rs17782313rs17066866rs1943226rs11875096rs1943224rs7235242rs11872992rs8093815rs17066856rs17066836rs1943227rs1943218rs17066829rs9966412rs17066859rs9965495rs12970134rs17700633rs11873305rs8091237rs7240064 | Energy homeostasis, appetite regulation | 95,96 |
PCSK1 | Proprotein convertase subtilisin/kexin type 1 | rs236918 | Insulin resistance | 97 |
PPARG | Peroxisome proliferator-activated receptor gamma | rs1801282 | Increased BMI | 98,99 |
ADBR2 | Adeno receptor beta2 | rs1042714rs1042713 | Adaptive thermogenesis | 100, 101 |
ADBR3 | Adeno receptor beta 3 | rs4994 | 102,103,104 | |
GHRL | Ghrelin | rs696217 | Appetite regulation | 105 |
FABP2 | Fatty acid binding protein 2 | rs1799883 | Fatty acid uptake | 107 |
APOA5 | Apolipoprotein A5 | rs964184rs662799 | Lipoprotein metabolism | 108,109 |
APOA1 | Apolipoprotein A1 | rs670 | 110 | |
LIPC | Lipase C hepatic type | rs2070895 | 111 | |
CETP | Cholesteryl ester transfer protein | rs3764261 | 112 | |
MTNR1B | Melatonin receptor 1B | rs10830963 | Appetite regulation | 113,114 |
NPY | Neuropeptide Y | rs16147 | 115 | |
GIPR | Gastric inhibitory polypeptide receptor | rs2287019 | Insulin signaling | 116 |
IRS1 | Insulin receptor substrate 1 | rs1522813rs2943641 | 117,118 | |
TCF7L2 | Transcrption factor 7 like 2 | rs12255372rs7903146 | Blood glucose homeostasis | 119 |
PCSK1 | Proprotein convertase subtilisin/Kexin type 1 | rs6232rs6234 | Energy metabolism | 120,121 |
Genes and physical activity
Physical activity levels and intensity play an important role in moderating the risk of weight gain, in both weight loss program and in prevention of weight regain (121). Physical activity is a modifiable life style and is associated with cardio-metabolic outcomes, including obesity, type 2 diabetes, and cardiovascular diseases (122,123). A recent study by Goryakin et al., reported that more than half of the Italian population does not meet the least moderate activity threshold set by world health organization despite the fact that the government has passed several health policies for the promotion of active transport, mass media campaigns, physical activity in primary care, school-based interventions and mobile apps in order to promote exercise and physical activity (124). Another study in 2015 has highlighted that only 20.8% of the US population meets the US national guidelines for physical activity while 50.8% of all adults do not meet those guidelines (125). In this context, approximately 35% of the mortality due to coronary heart disease has been attributed to insufficient physical activity (126), leading to the estimated $117 billion health care expenditures annually in the US (127).
Given the importance of physical activity many research reports based on twin studies (128), heritage analysis (129-134), linkage studies (135-139), genome wide association studies have been dedicated to unravel the genetic variants associated with physical activity (140-143). Genes that have been consistently associated with physical activity are FTO, ANKRD6, IL15R, PPARD, LEPR, CASR, PAPSS2, DRD2, GABRG3, ACE, MC4R (137, 144-151). The names, functions and notable variants of these genes have been listed in .
Table2.
Genes with their SNPs that influence metabolism of specific food and nutrients with a major impact on health status
Gene (OMIM ID) | Full name | SNP ID (risk allele) | SNP-associated phenotype | Diet intervention | Reference |
MCM6 (*601806) | Minichromosome maintenance complex component 6 | rs4988235 C>T (C)rs182549 G>A (G)rs145946881 G>C (G) | Lactose intolerance, adult type (#223100) | Avoid milk and its derivatives with high content of lactose | OMIM |
HLA-DQA1 (*146880) | Major histocompatibility complex, class II, DQ alpha 1 | rs2187668 C>T (C)rs2395182 G>T (G)rs4639334 G>A (G)rs4713586 A>G (G)rs7454108 T>C (C)rs7775228 T>C (C) | Susceptibility to celiac disease 1 (#212750) | Avoid foods containing gluten | |
HLA-DQB1 (*604305) | Major histocompatibility complex, class II, DQ beta 1 | Avoid foods containing gluten | |||
HJV (*608374) | Hemojuvelin BMP co-receptor | rs74315323 C>Ars74315324 G>Ars74315325 A>Trs74315326 A>Grs28940586 A>C,Grs74315327 A>Grs121434374 G>C,Trs786205063 (GA)3G>GAGrs121434375 T>A | Hemochromatosis, type 2A (#602390) | Avoid iron-rich foods | |
SLC40A1 (OMIM *604653) | Solute carrier family 40 member 1 | rs104893662 T>A,Grs28939076 G>Trs878854984 (CAA)4>(CAA)3,(CAA)5rs104893663 T>A,Crs104893670 C>A,Trs104893671 C>Ars104893672 T>Ars104893673 C>Ars104893664 C>T | Hemochromatosis, type 4 | ||
HFE (*613609) | Homeostatic iron regulator | rs1800562 G>Ars1799945 C>G,Trs1800730 A>Trs1800758 G>Ars28934889 G>Ars111033557 G>Ars28934595 A>Crs111033558 G>C,Trs28934596 T>Crs28934597 G>Crs111033563 A>C | Hemochromatosis | ||
TFR2 | Transferrin receptor 2 | rs80338880 G>Crs80338877 (G)5>(G)6rs80338879 A>Trs41303501 C>Trs80338889 T>C,G | Hemochromatosis, type 3 | OMIM | |
FTH1 | Ferritin heavy chain 1 | rs387906549 T>A | Hemochromatosis, type 5 | ||
HAMP (*606464) | Hepcidin antimicrobial peptide | Hemochromatosis, type 2B | |||
ADH1B (*103720) | Alcohol dehydrogenase 1B | rs1229984 A>Grs2066702 C>T | G/C, higher alcohol consumption.A/T, accumulation of acetaldehyde | Reduce the alcohol consumption | |
ADH1C (*103730) | Alcohol dehydrogenase 1C | rs1693482 C>T (T)rs698 T>A,C (C) | Type II alcoholism | ||
ALDH2 (*100650) | Aldehyde dehydrogenase 2 family member | rs671 G>A (A) | Acute alcohol sensitivity (#610251) | ||
CYP1A2 (+124060) | Cytochrome P450 family 1 subfamily A member 2 | rs762551 C>A (C) | Higher risk of nonfatal myocardial infarction | Reduce caffeine consumption | doi:10.1007/s00213-010-1900-1 |
ADORA2A (*102776) | Adenosine A2a receptor | rs5751876 T>C (C) | Greater caffeine sensitivity, sleep impairment, increased beta activity during non-REM sleep | ||
rs35320474 delT (T) | Greater caffeine-induced anxiety | ||||
DRD2 | Dopamine receptor D2 | rs1110976 T>G (G) | Greater caffeine-induced anxiety | ||
COMT | Catechol-O-methyltransferase | rs4680 G>A (A) | Higher risk of acute myocardial infarction | ||
ALDOB (*612724) | Fructose-bisphosphate B aldolase | rs1800546 C>G (G)rs76917243 G>T (T)rs118204425 AAGdel (del) | Fructose intolerance (#229600) | Reduce consumption of fruit and vegetables | OMIM |
UGT1A1 (*191740) | UDP glucuronosyltransferase family 1 member A1 | rs6742078 G>T (T) | Bilirubin serum level (#601816) | Reduce the consumption of proteins | |
G6PD (*305900) | Glucose-6-phosphate dehydrogenase | rs1050829 T>A,C (A) rs1050828 C>T (T) | Nonspherocytic hemolytic anemia (#300908) | Avoid the consumption of broad beans |
Table3.
Genes with their SNPs that influence metabolism of specific nutrients with minor effects on health status
Gene (OMIM ID) | Full name | SNP ID (risk allele) | SNP-associated phenotype | Diet intervention | Reference |
BCO1 | Beta-carotene oxygenase 1 | rs12934922 A>T (T) | Reduced conversion of beta-carotene to retinol | Increase the intake of beta-carotene | SNPedia |
rs7501331 C>T (T) | |||||
GC | GC Vitamin D Binding Protein | rs2282679 T>G (G) | Lower vitamin D levels | Increase consumpion of vitamin D-containing food | |
rs4588 G>T (T) | |||||
rs842999 C>G (C) | |||||
SLC23A1 | Solute carrier family 23 member 1 | rs33972313 C>T (T) | Reduction of circulating levels of vitamin C | Increase consumpion of vitamin C-containing food | doi:10.3945/ajcn.2010.29438 |
SLC30A8 | Solute carrier family 30 member 8 | rs11558471 A>G (G) | Susceptibility to diabetes mellitus | Reduce consumption of food with high content of carbohydrates | doi:10.1186/1471-2350-11-97 |
SLC5A6 | Solute carrier family 5 member 6 | rs1395 G>A (A) | Reduced intestinal uptake, cellular delivery and transplacental transport of pantothenate and biotin | Increase consumption of biotin- and pantothenate-containing food | doi:10.1111/jnc.13092 |
TCN2 | Transcobalamin 2 | rs1801198 C>G (G) | Decreased serum vitamin B12, increased homocysteine | Increase consumption of B12-containing food | SNPedia |
TTPA | Alpha tocopherol transfer protein | rs4501570 G>A (A) | Vitamin E deficiency | Increase consumption of vitam E-containing food | doi:10.3945/jn.112.160333 |
rs4587328 T>C (C) | |||||
rs4606052 C>T (T) | |||||
VDR | Vitamin D receptor | rs731236 A>G (G) | Immune weakness, increased cancer risk, early bone loss, increased cognitive decline risk, mood disorders | Increase consumption of vitam D-containing food | SNPedia |
CYP2R1 | Cytochrome P450 family 2 subfamily R member 1 | rs10741657 A>G (G) | Lower vitamin D levels | Increase consumption of food rich in vitamin D | OMIM |
rs10766197 A>G (A) | |||||
LPA | Lipoprotein(A) | rs10455872 A>G (G)rs3798220 C>T (C) | Coronary artery disease | Reduce trygliceride and cholesterol consumption | SNPedia |
Cardiovascular events risk | |||||
CDKN2B-AS1 | Cyclin dependent kinase inhibitor 2B-antisense RNA 1 | rs10757274 A>G (G)rs2383206 A>G (G)rs2383207 A>G (G) | Heart attack risk | ||
Intergenic | / | rs10757278 A>G (G) | Heart disease risk | ||
MC4R | Melanocortin 4 receptor | rs17782313 C>T (C) | Increased BMI | Reduce calorie intake | SNPedia |
APOA2 | Apolipoprotein A2 | rs5082 C>T (C) | Higher total energy, fat, protein intake | ||
PCSK1 | Proprotein convertase subtilisin/Kexin type 1 | rs6232 A>G (G) | Obesity and insulin sensitivity higher risk | ||
APOA5 | Apolipoprotein A5 | rs662799 A>G (G) | Early heart attack higher risk; less weight gain on high fat diets | ||
SH2B1 | SH2B adaptor protein 1 | rs7498665 A>G (G) | Obesity; type-2 diabetes | ||
SLC2A2 | Solute carrier family 2 member 2 | rs5400 C>T (T) | Higher sugar consumption | ||
F2 | Coagulation factor II, thrombin | rs1799963 A>G (A) | Thrombosis and cerebral stroke higher risk | Reduce tryglicerides and cholesterol consumption | |
F5 | Coagulation factor V | rs6025 A>G (A) | Thrombosis higher risk | ||
FUT2 | Fucosyltransferase 2 | rs602662 A>G (G) | Lower vitamin B12 levels | Increase consumption of food rich in vitamin B12 | |
ALPL | Biomineralization associated alkaline phosphatase | rs4654748 C>T (C) | Lower Vitamin B6 blood concentration | Increase consumption of food rich in vitamin B6 | |
CYP2R1 | Cytochrome P450 family 2 subfamily R member 1 | rs10741657 A>G (G)rs10766197 A>G (A) | Lower vitamin D levels | Increase consumption of food rich in vitamin D, increase sun exposure | |
GC | GC vitamin D binding protein | rs4588 G>T (T)rs842999 C>G (C) | |||
MTHFR (*607093) | Methylenetetrahydrofolate reductase | rs1801133 G>A (A) | Homocystinuria (#236250) | Increase folic acid intake | OMIM |
CBS (*613381) | Cystathionine beta synthase | rs121964962 C>T (T) | Homocystinuria (#236200) | ||
FOXO3 | Forkhead box O3 | rs2802292 C>T (T) | Longer lifespan | SNPedia | |
rs2802288 A>G (A) | |||||
rs2802292 T>G (G) | |||||
SIRT1 | Sirtuin 1 | rs3740051 | Higher basal energy expenditure | doi:10.1371/journal.pone.0058636 | |
rs2236319 | |||||
rs2272773 | |||||
PEMT | Phosphatidylethanolamine N-methyltransferase | rs12325817 G>C (C) | Low choline | Increase choline intake | doi:10.1096/fj.06-5734com |
CHDH | Choline dehydrogenase | rs12676 G>T (T) |
A cross talk between genes, diet and physical activity for weight management
Genetic polymorphisms linked to obesity may exert their effects by influencing physical activity and, on the other hand, physically active life style may reduce the risk of obesity. Current evidences have shown that physical activity can modulate heritability estimates for obesity-related traits (125). For instance a study revealed significantly strong interactions between FTO intron 1 and physical activity in 16 independent cross sectional and interventional studies carried out in adults and children with European, African and East African origin (19, 127). In addition to this, a recent meta-analysis in individuals of European ancestry (sample size: 111421) proved a significant physical activity-genetic risk score (GRS) interaction with respect to 12 obesity predisposing polymorphism. The study indicated that this physical activity-GRS association is more evident in subjects living in North America (60).
Another meta-analysis on 218166 adults and 19268 children revealed that greater leisure time physical activity attenuated the effects of FTO variants, however, prolonged sedentary periods, such as watching TV, enhanced genetic predisposition to elevated adiposity (152). Moreover, Rankinen et al., reported that genetic association of FTO with BMI was strengthened by prolonged sedentary periods of watching TV in both men and women (153). Similar finding were reported by in another UK Biobank study with large sample size (154). They reported a strong genetic association with sedentary life style and risk of obesity which was worsened by inadequate sleep (154). The Diabetes Prevention Program in the US reported a strong interaction between FTO and a 1-y lifestyle intervention regime of physical activity, diet, and weight loss with respect to subcutaneous fat area among 869 individuals (155). They found the minor allele of the FTO variant is associated with increased subcutaneous fat area in the control group as compared to the lifestyle intervention group (155). Similarly a recent study indicated that physical activity combined with vegetarian diet can reduce the increased BMI caused by susceptibility due to FTO rs3751812 minor allele (156). Other physical activity-associated genes that can respond to dietary intake are genes involved in muscle structure and strength. A study in 461 European American adults showed the association of ACE I/D polymorphism rs4340 with a weekly walking distance of approximately 8 Km, ultimately reducing the BMI (157).
The aforementioned studies are a few examples of cross talk between genetic factors governing obesity and physical exercise. It can be concluded here that although genetic factors predispose to increased risk of obesity the environmental, behavioral, socioeconomic, psychological factors that contribute to overall life style determine the overall phenotype of an individual. Physical activity in this regard plays a vital role. Several studies have shown the importance of diet coupled with physical activity in maintaining a healthy weight. However there is no such one size fit for all diet that can meet the dietary requirements of people belonging to various ethnic groups that keeps the obesity at bay without the need of physical activity.
Commercial genetic testing for personalized diet recommendations
Two types of genetic tests are basically carried out in commercial settings i.e. laboratory developed tests and direct to consumer tests (DTC). Both of these tests are used to identify genetic factors contributing to health related issues and to provide dietary recommendations. The DTC testing industry provides individuals an easy access to their own genetic information. Many of these tests aim at informing the susceptibility to different diseases while some deliver nutrition recommendations (1). As a result, supporters of this technology assert that the information provided by these tests may result in a positive impact on health behaviors such as diet, smoking and exercise thus preventing the development of chronic diseases. However, unregulated nature of the industry is a continuous source of controversies surrounding DTC genetic testing.
The major requirement of a nutrigenetic test is that the outcomes must specify a diet-related recommendation that is advantageous for individuals. In addition to that, the results should be based on evidences that have been replicated and give consistent results. Inferences should be drawn based on realistic approaches. Ethical issues should be addressed accordingly.
Ethical concerns related to commercial genetic testing
Keeping in view the quality of genetic profiling tests and their consequent impacts on human life, these tests are prone to several ethical issues related to human welfare, sensitivity of such information and psychological effects of test outcomes. Nearly two million genetic test panels are commercially available with different companies analyzing different genetic polymorphisms (158,159).
Dietary recommendations based on genetic tests that give inconclusive or unreliable information may result in unwarranted limitations and issues (160).
In addition, the interpretation of these results is very important. Only a highly trained health professional having an in-depth knowledge of gene-diet-environment interactions should actually be allowed to interpret the results and extend recommendations . This implicates the need of education in the nutrigenetic, nutrigenomic and nutri-epigenomic disciplines and such courses should be included in curriculum (161).
Guidelines and legal regulations for genetic testing and personalised nutrition
The main aim for the guidelines and regulation for genetic test is to protect individuals from the services that can harm the individual physically, sentimentally or psychologically and to safeguard their rights. For genetic testing and personalized nutrition the regulations are mostly pertaining to analytical methods, clinical validity and clinical utility of the results. Due to increased trend of nutrigenetic research and its practical applications in commercial perspective several public, private organizations have developed guidelines for ensuring safety and well-being of human subjects that are either involved in the studies or are consumers of DTC and dietary prescriptions. European nutrigenomics organization (NuGO) established guidelines for the nutrition genomic research in 2007 (162). These guidelines were formulated based on the international laws safeguarding basic human rights. These specifically mention the importance of informed consent for participation of human subjects in any of the nutrigenetics or nutrigenomic trials or observation studies. However these guidelines are not law so every research involving human subjects should abide by the local legislations of that area. NuGO has highlighted the ethical issue related to genetic testing and consequent release of results for any studies related to nutrigenomics. According to the guidelines all possible measures should be taken in disclosing the results of genetic testing to individuals. For instance it is stated that: Genotype results attained during nutrigenomics research should preferably be communicated to the research volunteers on a study group level, and should not be disclosed individually. The reason being scarce scientific evidence linking specific genetic variant to diet sensitive pathological condition or response to any dietary intervention. Besides that phenotypic expression of a particular genotype is influenced by many confounding factors hence it is very difficult to be interpreted with respect to health risks and benefits for a particular individual. Moreover disclosing the results of epidemiological studies with large number of subjects could possibly convert the studies into mass screening which may give rise to different budgeting and counselling implications (162). In case of test result disclosure to individuals NuGO advices to convey the results through highly trained professionals who can answer any questions related to outcome of results and detailed written results should also be given in case the consultation by Physician or health care provider is required.
In addition to organizations like NuGO, the government organizations and the agencies of various countries have published rules regulating DTC tests which help to explain, inform and aware individuals. In USA there are three agencies regulating the genetic tests: FDA (Food and Drug Administration), CMS (Centers for medical and Medicaid services), FTC (Federal trade commission) (163). Furthermore, EU also issued a paper in 2002 based on the guidelines of genetic tests that addresses patients privileges in several European countries, mainly concerning to the right to acquire information, confidentiality, privacy and informed consent (164). The human genetics commission (HGC) in UK published a paper in 2010 on ethical guidelines for the genetic tests including the transparency, availability, ease of getting information and standardized testing procedures (165). Similarly the German governments implemented instructions associated to the authorization of laboratories, the necessity for well-versed agreement and genetic counselling (166). While there are no particular regulation for DTC tests in Belgium and Netherland yet there is a law stating that compelling the involvement of medical physician DTC tests of a medical nature in Belgium (167) and obtaining a permit from the Dutch Minister of welfare and sports in the Netherlands for some genetic tests (168). Whereas the department of health of the Australian government decreed that that testing should guarantee safety and quality and forbids the sale of DTC tests for severe diseases However the regulatory framework in Europe, Food4Me however concluded that neither EU nor its associate states have legal instruments specially dealing with personalized nutrition (169,170).
Controversies related to commercial genetic testing and personalised nutrition
Several controversies are in the air because of rapid increase in genetic testing services aimed at providing tailor made fancy diets and specifically because of some of web based companies and their fraudulent claims. Besides a research boom in the field of nutrigenetic the authenticity of genetic basis of DTCs and the dietary recommendations are still debatable. This is particularly due to the reason that where majority of nutrigenomic studies are thriving to find an evidence of association of genetic variants, polymorphisms and mutations with dietary/ metabolic phenotypes, a considerable amount of observational studies and trials based on large cohorts of human subjects have proven no association. Notable examples in this regard are the PREDICT study involving 700 twins and 400 non twins. Their preliminary results presented at the 2019 meeting of the American Society of Nutrition, confirmed that Different people respond very differently to the same dietary inputs and there is no one dietary approach thats works best for everyone (171). The differences in dietary responses in this study were attributed more to factors such as sleep habits, exercise, stress and gut microbes than to genetics. Another important study DIETFIT concluded that there were no significant differences in weight loss pattern among subjects undertaking diets matched vs unmatched with their genotypes. Moreover there was gene-diet interactions for waist circumference, BMI and body fat percentage (172). Besides these social aspects of personalised nutrition related to an increased healthism and medicalisation of diet are also continuous source of controversy (173).
Conclusion
The advancement in science and availability of technologies like, genomics, transcriptomics, proteomics, metabolomics coupled with information derived from The Human Genome Project has opened the doors for personalised interventions in diet to address metabolic issues related to diseases, disorders and behavioral patterns. Although genes have been found culprit in epidemics like obesity and sedentation, social, moral, psychological and other confounding environmental and epigenetic factors may favor or oppose a typical dietary response. Physical activity holds promise in this regard and adding moderate physical activity to daily life routines coupled with a balanced diet help achieve a desired weight loss goal. Despite of the challenges faced by the nutrigenomics and personalized nutrition in terms of scarcity of scientific evidence, technological barriers, confidentiality and validity of genetic testing, and ethical concerns related to humans both at nutrigenetic services provision and receiving end, this fields holds promise in improving the quality of human life by interventions complementing, silencing or enhancing the expression of our genes.
Conflict of interest:
Each author declares that he or she has no commercial associations (e.g. consultancies, stock ownership, equity interest, patent/licensing arrangement etc.) that might pose a conflict of interest in connection with the submitted article
References
1.
Ferguson LR, De Caterina R, Grman U, et al. Guide and position of the international society of nutrigenetics/nutrigenomics on personalised nutrition: part 1 - Fields of precision nutrition. J Nutrigenet Nutrigenomics. 2016;9:1227. [PubMed] [Google Scholar]2.
Kohlmeier M, De Caterina R, Ferguson LR, et al. Guide and position of the international society of nutrigenetics/nutrigenomics on personalized nutrition: part 2 - Ethics, challenges and endeavors of precision nutrition. J Nutrigenet Nutrigenomics. 2016;9:2846. [PubMed] [Google Scholar]3.
Simopoulos AP. Nutrigenetics/nutrigenomics. Annu Rev Public Health. 2010;31:5368. [PubMed] [Google Scholar]5.
Trujillo E, Davis C, Milner J. Nutrigenomics, proteomics, metabolomics, and the practice of dietetics. J Am Diet Assoc. 2006;106:40313. [PubMed] [Google Scholar]6.
Ferguson LR. Nutrigenomics approaches to functional foods. J Am Diet Assoc. 2009;109:4528. [PubMed] [Google Scholar]7.
Kaput J. Nutrigenomics research for personalized nutrition and medicine. Curr Opin Biotechnol. 2008;19:11020. [PubMed] [Google Scholar]8.
Ordovas JM, Corella D. Nutritional genomics. Annu Rev Genomics Hum Genet. 2004;5:71118. [PubMed] [Google Scholar]9.
Fenech MF. Nutriomes and nutrient arrays the key to personalised nutrition for DNA damage prevention and cancer growth control. Genome Integr. 2010;1:11. [PMC free article] [PubMed] [Google Scholar]10.
Simopoulos AP, Ordovas JM. World Review of Nutrition and Dietetics. Nutrigenetics and Nutrigenomics, Karger, Basel. 2004 [Google Scholar]11.
Frazer KA, Murray SS, Schork NJ, Topol EJ. Human genetic variation and its contribution to complex traits. Nat Rev Genet. 2009;10:24151. [PubMed] [Google Scholar]12.
Ordovas JM. Gender, a significant factor in the cross talk between genes, environment, and health. Gend Med. 2007;4:S11122. [PubMed] [Google Scholar]15.
Frayling TM, Timpson NJ, Weedon MN, et al. A common variant in the FTO gene is associated with body mass index and predisposes to childhood and adult obesity. Science. 2007;316:88994. [PMC free article] [PubMed] [Google Scholar]16.
Farooqi IS, ORahilly S. Genetics of obesity in humans. Endocr Rev. 2006;10:7108. [PubMed] [Google Scholar]17.
Martinez JA, Parra MD, Santos JL, Moreno-Aliaga MJ, Marti A, Martinez-Gonzalez MA. Genotype-dependent response to energy-restricted diets in obese subjects: towards personalized nutrition. Asia Pacific J Clin Nutr. 2008;17:11922. [PubMed] [Google Scholar]18.
Razquin C, Marti A, Martinez JA. Evidences on three relevant obesogenes: MC4R, FTO and PPARgamma. Approaches for personalized nutrition. Mol Nutr Food Res. 2011;55:13649. [PubMed] [Google Scholar]19.
Wang J, Wang LJ, Zhong Y, et al. CETP gene polymorphisms and risk of coronary atherosclerosis in a Chinese population. Lipids Health Disease. 2013;12:176. [PMC free article] [PubMed] [Google Scholar]20.
Lu Y, Tayebi N, Li H, Saha N, Yang H, Heng CK. Association of CETP Taq1B and-629C> A polymorphisms with coronary artery disease and lipid levels in the multi-ethnic Singaporean population. Lipids Health Disease. 2013;12:85. [PMC free article] [PubMed] [Google Scholar]21.
Huang D, Xie X, Ma YT, Huang Y, Ma X. Endothelial lipase-384A/C polymorphism is associated with acute coronary syndrome and lipid status in elderly Uygur patients in Xinjiang. Genet Testing Mol Biomarkers. 2014;18:7814. [PMC free article] [PubMed] [Google Scholar]22.
Knoblauch H, Bauerfeind A, Krahenbuhl C, et al. Common haplotypes in five genes influence genetic variance of LDL and HDL cholesterol in the general population. Hum Mol Genet. 2002;11:147785. [PubMed] [Google Scholar]23.
Lyssenko V, Lupi R, Marchetti P, et al. Mechanisms by which common variants in the TCF7L2 gene increase risk of type 2 diabetes. J Clin Invest. 2007;117:215563. [PMC free article] [PubMed] [Google Scholar]25.
Mirabello L, Huang WY, Wong JY, et al. The association between leukocyte telomere length and cigarette smoking, dietary and physical variables, and risk of prostate cancer. Aging Cell. 2009;8:40513. [PMC free article] [PubMed] [Google Scholar]26.
Liew SC, Gupta ED. Methylenetetrahydrofolate reductase (MTHFR) C677T polymorphism: epidemiology, metabolism and the associated diseases. Eur J Med Genet. 2015;58:110. [PubMed] [Google Scholar]27.
Cornelis MC, El-Sohemy A, Kabagambe EK, et al. Coffee, CYP1A2 genotype, and risk of myocardial infarction. JAMA. 2006;295:113541. [PubMed] [Google Scholar]28.
Corella D, Peloso G, Arnett DK, et al. APOA2, dietary fat, and body mass index: replication of a gene-diet interaction in 3 independent populations. Arch Intern Med. 2009;169:1897906. [PMC free article] [PubMed] [Google Scholar]29.
Corella D, Tai ES, Sorl JV, et al. Association between the APOA2 promoter polymorphism and body weight in Mediterranean and Asian populations: replication of a gene-saturated fat interaction. Int J Obes (Lond) 2011;35:66675. [PMC free article] [PubMed] [Google Scholar]30.
Cahill LE, Fontaine-Bisson B, El-Sohemy A. Functional genetic variants of glutathione S-transferase protect against serum ascorbic acid deficiency. Am J Clin Nutr. 2009;90:14117. [PubMed] [Google Scholar]31.
Slater NA, Rager ML, Havrda DE, et al. Genetic variation in CYP2R1 and GC genes associated with vitamin D deficiency status. J Pharm Pract. 2017;30:316. [PubMed] [Google Scholar]32.
Tanwar VS, Chand MP, Kumar J, et al. Common variant in FUT2 gene is associated with levels of vitamin B(12) in Indian population. Gene. 2013;515:2248. [PubMed] [Google Scholar]33.
Benyamin B, Ferreira MA, Willemsen G, et al. Common variants in TMPRSS6 are associated with iron status and erythrocyte volume. Nat Genet. 2009;41:11735. [PMC free article] [PubMed] [Google Scholar]35.
Nielsen DE, El-Sohemy A. Disclosure of genetic information and change in dietary intake: a randomized controlled trial. PLoS One. 2014;9:e112665. [PMC free article] [PubMed] [Google Scholar]36.
De Toro-Martin J, Arsenault BJ, Despres JP, Vohl MC. Precision nutrition: a review of personalized nutritional approaches for the prevention and management of metabolic syndrome. Nutrients. 2017;9:913. [PMC free article] [PubMed] [Google Scholar]37.
Nizel AE. Personalized nutrition counseling. ASDC J Dent Child. 1972;39:35360. [PubMed] [Google Scholar]38.
Brug J, Campbell M, van Assema P. The application and impact of computer-generated personalized nutrition education: A review of the literature. Patient Educ Couns. 1999;36:14556. [PubMed] [Google Scholar]39.
Stewart-Knox B, Kuznesof S, Robinson J, et al. Factors influencing European consumer uptake of personalised nutrition. Results of a qualitative analysis. Appetite. 2013;66:6774. [PubMed] [Google Scholar]40.
Wang DD, Hu FB. Precision nutrition for prevention and management of type 2 diabetes. Lancet Diabetes Endocrinol. 2018;6:41626. [PubMed] [Google Scholar]42.
Grimaldi KA, van Ommen B, Ordovas JM, et al. Proposed guidelines to evaluate scientific validity and evidence for genotype-based dietary advice. Genes Nutr. 2017;12:35. [PMC free article] [PubMed] [Google Scholar]43.
Bouteldja N, Timson DJ. The biochemical basis of hereditary fructose intolerance. J Inherit Metab Dis. 2010;33:10512. [PubMed] [Google Scholar]44.
de Baulny HO, Abadie V, Feillet F, de Parscau L. Management of phenylketonuria and hyperphenylalaninemia. J Nutr. 2007;137:1561S3S. [PubMed] [Google Scholar]45.
Novelli G, Reichardt JK. Molecular basis of disorders of human galactose metabolism: past, present, and future. Mol Genet Metab. 2000;71:625. [PubMed] [Google Scholar]46.
Heap GA, van Heel DA. Genetics and pathogenesis of coeliac disease. Semin Immunol. 2009;21:34654. [PubMed] [Google Scholar]48.
Fallaize R, Macready AL, Butler LT, Ellis JA, Lovegrove JA. An insight into the public acceptance of nutrigenomic-based personalised nutrition. Nutr Res Rev. 2013;26:3948. [PubMed] [Google Scholar]49.
Atkinson SA. Defining the process of dietary reference intakes: frameworkfor the United States and Canada. Am J Clin Nutr. 2011;94:655S7S. [PMC free article] [PubMed] [Google Scholar]51.
Timotijevic L, Brown KA, Lhteenmki L, et al. EURRECAa framework for considering evidence in public health nutrition policy development. Crit Rev Food Sci Nutr. 2013;53:112434. [PubMed] [Google Scholar]52.
Claessens M, Contor L, Dhonukshe-Rutten R, et al. EURRECAprinciples and future for deriving micronutrient recommendations. Crit Rev Food Sci Nutr. 2013;53:113546. [PubMed] [Google Scholar]55.
Brunkwall L, Chen Y, Hindy G, et al. Sugar-sweetened beverage consumption and genetic predisposition to obesity in 2 Swedish cohorts. Am J Clin Nutr. 2016;104:80915. [PMC free article] [PubMed] [Google Scholar]56.
Olsen NJ, Angquist L, Larsen SC, et al. Interactions between genetic variants associated with adiposity traits and soft drinks in relation to longitudinal changes in body weight and waist circumference. Am J Clin Nutr. 2016;104:81626. [PubMed] [Google Scholar]57.
Qi Q, Chu AY, Kang JH, et al. Fried food consumption, genetic risk, and body mass index: Gene-Diet interaction analysis in three us cohort studies. BMJ. 2014;348:g1610. [PMC free article] [PubMed] [Google Scholar]58.
Ahmad S, Rukh G, Varga TV, et al. Gene physical activity interactions in obesity: Combined analysis of 111,421 individuals of european ancestry. PLoS Genet. 2013;9:e1003607. [PMC free article] [PubMed] [Google Scholar]59.
Tyrrell J, Wood AR, Ames RM, et al. Gene-obesogenic environment interactions in the UK biobank study. Int J Epidemiol. 2017;46:55975. [PMC free article] [PubMed] [Google Scholar]60.
Alfredo Martnez J. Perspectives on personalized nutrition for obesity. J Nutrigenet Nutrigenomics. 2014;7:IIII. [PubMed] [Google Scholar]61.
Marreiro Ddo N, de Sousa AF, Nogueira Ndo N, Oliveira FE. Effect of zinc supplementation on thyroid hormone metabolism of adolescents with Down syndrome. Biol Trace Elem Res. 2009;129:207. [PubMed] [Google Scholar]62.
Pogribna M, Melnyk S, Pogribny I, Chango A, Yi P, James SJ. Homocysteine metabolism in children with Down syndrome: in vitro modulation. Am J Hum Genet. 2001;69:8895. [PMC free article] [PubMed] [Google Scholar]63.
Ordovas JM, Mooser V. Nutrigenomics and nutrigenetics. Curr Opin Lipidol. 2004;15:1018. [PubMed] [Google Scholar]64.
Rao AD, Sun B, Saxena A, et al. Polymorphisms in the serum-and glucocorticoid-inducible kinase 1 gene are associated with blood pressure and renin response to dietary salt intake. J Hum Hypertens. 2013;27:17680. [PMC free article] [PubMed] [Google Scholar]65.
Mustalahti K, Catassi C, Reunanen A, et al. The prevalence of celiac disease in Europe: results of a centralized, international mass screening project. Ann Med. 2010;42:58795. [PubMed] [Google Scholar]66.
Fenech M, El-Sohemy A, Cahill L, et al. Nutrigenetics and nutrigenomics: viewpoints on the current status and applications in nutrition research and practice. J Nutrigenet Nutrigenomics. 2011;4:6989. [PMC free article] [PubMed] [Google Scholar]67.
Burkhardt R, Kirsten H, Beutner F, et al. Integration of genome-wide SNP data and gene-expression profiles reveals six novel loci and regulatory mechanisms for amino acids and acylcarnitines in whole blood. PLoS Genet. 2015;11:e1005510. [PMC free article] [PubMed] [Google Scholar]68.
Goni L, Cuervo M, Milagro FI, Martnez A. Future perspectives of personalized weight loss interventions based on nutrigenetic, epigenetic, and metagenomic data. J Nutr. 2015;146:905S12S. [PubMed] [Google Scholar]69.
Precone V, Beccari T, Stuppia L, et al. Taste, olfactory and texture related genes and food choices: Implications on health status. Eur Rev Med Pharmacol Sci. 2019;23:130521. [PubMed] [Google Scholar]70.
Grimaldi K. Nutrigenetics and personalized nutrition: Are we ready for DNA-based dietary advice? Pers Med. 2014;11:297307. [PubMed] [Google Scholar]71.
De Caterina R, El-Sohemy A. Moving towards specific nutrigenetic recommendation algorithms: caffeine, genetic variation and cardiovascular risk. J Nutr Nutr. 2016;9:10615. [PubMed] [Google Scholar]72.
Frayling TM, Timpson NJ, Weedon M, et al. A common variant in the FTO gene is associated with body mass index and predisposes to childhood and adult obesity. Science. 2007;316:88994. [PMC free article] [PubMed] [Google Scholar]73.
Hetherington MM, Cecil JE. Gene-environment interactions in obesity. Forum Nutr. 2009;63:195203. [PubMed] [Google Scholar]74.
Ortega-Azorn C, Sorl JV, Asensio EM, et al. Associations of the FTO rs9939609 and the MC4R rs17782313 polymorphisms with type 2 diabetes are modulated by diet, being higher when adherence to the Mediterranean diet pattern is low. Cardiovasc Diabetol. 2012;11:137. [PMC free article] [PubMed] [Google Scholar]75.
Fisher E, Boeing H, Fritsche A, Doering F, Joost HG, Schulze MB. Whole-grain consumption and transcription factor-7-like 2 (TCF7L2) rs7903146: Genediet interaction in modulating type 2 diabetes risk. Br J Nutr. 2008;101:47881. [PubMed] [Google Scholar]76.
Hindy G, Sonestedt E, Ericson U, et al. Role of TCF7L2 risk variant and dietary fibre intake on incident type 2 diabetes. Diabetologia. 2012;55:264654. [PMC free article] [PubMed] [Google Scholar]77.
Wirstrm T, Hilding A, Gu HF, Ostenson CG, Bjrklund A. Consumption of whole grain reduces risk of deteriorating glucose tolerance, including progression to prediabetes. Am J Clin Nutr. 2012;97:17987. [PubMed] [Google Scholar]78.
Lpez-Ortiz MM, Garay-Sevilla ME, Tejero ME, Prez-Luque E. Analysis of the interaction between transcription factor 7-like 2 genetic variants with nopal and wholegrain fibre intake: Effects on anthropometric and metabolic characteristics in type 2 diabetes patients. Br J Nutr. 2016;116:96978. [PubMed] [Google Scholar]79.
Franzago M, Fraticelli F, Nicolucci A, et al. Molecular analysis of a genetic variants panel related to nutrients and metabolism: association with susceptibility to gestational diabetes and cardiometabolic risk in affected women. J Diabetes Res 2017. 2017:4612623. [PMC free article] [PubMed] [Google Scholar]80.
Franzago M, Fraticelli F, Marchetti D, et al. Nutrigenetic variants and cardio-metabolic risk in women with or without gestational diabetes. Diabetes Res Clin Pract. 2018;137:6471. [PubMed] [Google Scholar]81.
Franzago M, Fraticelli F, Di Nicola M, et al. Early subclinical atherosclerosis in gestational diabetes: the predictive role of routine biomarkers and nutrigenetic variants. J Diabetes Res 2018. 2018:9242579. [PMC free article] [PubMed] [Google Scholar]82.
Dhurandhar NV, Schoeller D, Brown AW, et al. Energy balance measurement: When something is not better than nothing. Int J Obes. 2014;39:110913. [PMC free article] [PubMed] [Google Scholar]83.
Archer E, Marlow ML, Lavie CJ. Controversy and debate: memory based methods paper 3: nutritions Black Swans: Our reply. J Clin Epidemiol. 2018;104:1305. [PubMed] [Google Scholar]84.
Matsuo T, Nakata Y, Murotake Y, Hotta K, Tanaka K. Effects of FTO genotype on weight loss and metabolic risk factors in response to calorie restriction among Japanese women. Obesity (Silver Spring) 2012;20:11226. [PubMed] [Google Scholar]85.
Zheng Y, Huang T, Zhang X, et al. Dietary fat modifies the effects of FTO genotype on changes in insulin sensitivity. J Nutr. 2015;145:97782. [PMC free article] [PubMed] [Google Scholar]86.
Grau K, Hansen T, Holst C, et al. Macronutrient-specific effect of FTO rs9939609 in response to a 10-week randomized hypo-energetic diet among obese Europeans. Int J Obes (Lond) 2009;33:122734. [PubMed] [Google Scholar]87.
Zhang X, Qi Q, Zhang C, et al. FTO genotype and 2-year change in body composition and fat distribution in response to weight-loss diets: the POUNDS LOST Trial. Diabetes. 2012;61:300511. [PMC free article] [PubMed] [Google Scholar]88.
Mitchell JA, Church TS, Rankinen T, Earnest CP, Sui X, Blair SN. FTO genotype and the weight loss benefits of moderate intensity exercise. Obesity (Silver Spring) 2010;18:6413. [PMC free article] [PubMed] [Google Scholar]89.
Labayen I, Margareto J, Maldonado-Martin S, et al. Independent and combined influence of the FTO rs9939609 and MC4Rrs17782313 polymorphisms on hypocaloric diet induced changes in body mass and composition and energy metabolism in non-morbid obese premenopausal women. Nutr Hosp. 2015;31:202532. [PubMed] [Google Scholar]90.
De Luis DA, Izaola O, Primo D, et al. Role of rs1501299 variant in the adiponectin gene on total adiponectin levels, insulin resistance and weight loss after a Mediterranean hypocaloric diet. Diabetes Res Clin Pract. 2019;148:2627. [PubMed] [Google Scholar]91.
Zhang Y, Proenca R, Maffei M, Barone M, Leopold L, Friedman JM. Positional cloning of the mouse obese gene and its human homologue. Nature. 1994;372:42532. [PubMed] [Google Scholar]92.
De Luis Roman D, de la Fuente RA, Sagrado MG, Izaola O, Vicente RC. Leptin receptor Lys656Asn polymorphism is associated with decreased leptin response and weight loss secondary to a lifestyle modification in obese patients. Arch Med Res. 2006;37:8549. [PubMed] [Google Scholar]93.
Walley AJ, Asher JE, Froguel P. The genetic contribution to non-syndromic human obesity. Nat Rev Genet. 2009;10:43142. [PubMed] [Google Scholar]95.
Labayen I, Margareto J, Maldonado-Martin S, et al. Independent and combined influence of the FTO rs9939609 and MC4R rs17782313 polymorphisms on hypocaloric diet induced changes in body mass and composition and energy metabolism in non-morbid obese premenopausal women. Nutr Hosp. 2015;31:202532. [PubMed] [Google Scholar]96.
Pan Q, Delahanty LM, Jablonski KA, et al. Variation at the melanocortin 4 receptor gene and response to weight-loss interventions in the diabetes prevention program. Obesity (Silver Spring) 2013;21:E5206. [PMC free article] [PubMed] [Google Scholar]97.
Huang T, Huang J, Qi Q, et al. PCSK7genotype modifies effect of a weight-loss diet on 2-year changes of insulin resistance: The pounds lost trial. Diabetes Care. 2015;38:43944. [PMC free article] [PubMed] [Google Scholar]98.
Tonjes A, Scholz M, Loeffler M, Stumvoll M. Association of Pro12Ala polymorphism in peroxisome proliferator-activated receptor gamma with pre-diabetic phenotypes: meta-analysis of 57 studies on nondiabetic individuals. Diabetes Care. 2006;29:248997. [PubMed] [Google Scholar]99.
Meirhaeghe A, Amouyel P. Impact of genetic variation of PPAR gamma in humans. Mol Genet Metab. 2004;83:93102. [PubMed] [Google Scholar]100.
Ruiz JR, Larrarte E, Margareto J, Ares R, Labayen I. Role of 2-adrenergic receptor polymorphisms on body weight and body composition response to energy restriction in obese women: preliminary results. Obesity (Silver Spring) 2011;19:2125. [PubMed] [Google Scholar]101.
Saliba LF, Reis RS, Brownson RC, et al. Obesity-related gene ADRB2, ADRB3 and GHRL polymorphisms and the response to a weight loss diet intervention in adult women. Genet Mol Biol. 2014;37:1522. [PMC free article] [PubMed] [Google Scholar]102.
Gardner CD, Trepanowski JF, Del Gobbo LC, et al. Effect of low-fat vs low-carbohydrate diet on 12-Month weight loss in overweight adults and the association with genotype pattern or insulin secretion: the DIETFITS randomized clinical trial. JAMA. 2018;319:66779. [PMC free article] [PubMed] [Google Scholar]103.
Tahara A, Osaki Y, Kishimoto T. Effect of the 3-adrenergic receptor gene polymorphism Trp64Arg on BMI reduction associated with an exercise-based intervention program in Japanese middle-aged males. Environ Health Prev Med. 2010;15:3927. [PMC free article] [PubMed] [Google Scholar]104.
Shiwaku K, Nogi A, Anuurad E, et al. Difficulty in losing weight by behavioral intervention for women with Trp64Arg polymorphism of the beta3-adrenergic receptor gene. Int J Obes Relat Metab Disord. 2003;27:102836. [PubMed] [Google Scholar]105.
Korbonits M, Gueorguiev M, OGrady E, et al. A variation in the ghrelin gene increases weight and decreases insulin secretion in tall, obese children. J Clin Endocrinol Metab. 2002;87:40058. [PubMed] [Google Scholar]106.
Zarebska A, Jastrzebski Z, Cieszczyk P, et al. The Pro12Ala polymorphism of the peroxisome proliferator-activated receptor gamma gene modifies the association of physical activity and body mass changes in Polish women. PPAR Res 2014. 2014:373782. [PMC free article] [PubMed] [Google Scholar]107.
Mocking RJT, Lok A, Assies J, et al. Ala54Thr fatty acid-binding protein 2 (FABP2) polymorphism in recurrent depression: associations with fatty acid concentrations and waist circumference. PLoS One. 2012;8:e82980. [PMC free article] [PubMed] [Google Scholar]108.
Zhang X, Qi Q, Bray GA, Hu FB, Sacks FM, Qi L. APOA5 genotype modulates 2-y changes in lipid profile in response to weight-loss diet intervention: the Pounds Lost Trial. Am J Clin Nutr. 2012;96:91722. [PMC free article] [PubMed] [Google Scholar]109.
Suchanek P, Lorenzova A, Poledne R, Hubacek JA. Changes of plasma lipids during weight reduction in females depends on APOA5 variants. Ann Nutr Metab. 2008;53:1048. [PubMed] [Google Scholar]110.
De Luis DA, Izaola O, Primo D, Aller R. Role of rs670 variant of APOA1 gene on lipid profile, insulin resistance and adipokine levels in obese subjects after weight loss with a dietary intervention. Diabetes Res Clin Pract. 2018;142:13945. [PubMed] [Google Scholar]111.
Xu M, Ng SS, Bray GA, et al. Dietary fat intake modifies the effect of a common variant in the LIPC gene on changes in serum lipid concentrations during a long-term weight-loss intervention trial. J Nutr. 2015;145:128994. [PMC free article] [PubMed] [Google Scholar]112.
Qi Q, Durst R, Schwarzfuchs D, et al. CETP genotype and changes in lipid levels in response to weight loss diet intervention in the POUNDS LOST and DIRECT randomized trials. J Lipid Res. 2015;56:71321. [PMC free article] [PubMed] [Google Scholar]113.
De Luis DA, Izaola O, Primo D, Aller R. Association of the rs10830963 polymorphism in melatonin receptor type 1B (MTNR1B) with metabolic response after weight loss secondary to a hypocaloric diet based in Mediterranean style. Clin Nutr. 2018;37:15638. [PubMed] [Google Scholar]114.
Goni L, Sun D, Heianza Y, et al. Macronutrient-specific effect of the MTNR1B genotype on lipid levels in response to 2 year weight-loss diets. J Lipid Res. 2018;59:15561. [PMC free article] [PubMed] [Google Scholar]115.
Lin X, Qi Q, Zheng Y, et al. Neuropeptide Y genotype, central obesity, and abdominal fat distribution: the POUNDS LOST trial. Am J Clin Nutr. 2015;102:5149. [PMC free article] [PubMed] [Google Scholar]116.
Qi Q, Bray GA, Hu FB, Sacks FM, Qi L. Weight-loss diets modify glucose-dependent insulinotropic polypeptide receptor rs2287019 genotype effects on changes in body weight, fasting glucose, and insulin resistance: the Preventing Overweight Using Novel Dietary Strategies trial. Am J Clin Nutr. 2012;95:50613. [PMC free article] [PubMed] [Google Scholar]117.
Qi Q, Bray GA, Smith SR, Hu FB, Sacks FM, Qi L. Insulin receptor substrate 1 gene variation modifies insulin resistance response to weight-loss diets in a 2-year randomized trial: the Preventing Overweight Using Novel Dietary Strategies (POUNDS LOST) trial. Circulation. 2011;124:56371. [PMC free article] [PubMed] [Google Scholar]118.
Qi Q, Xu M, Wu H, et al. IRS1 genotype modulates metabolic syndrome reversion in response to 2-year weight-loss diet intervention: the POUNDS LOST trial. Diabetes Care. 2013;36:34427. [PMC free article] [PubMed] [Google Scholar]119.
Grau K, Cauchi S, Holst C, et al. TCF7L2 rs7903146macronutrient interaction in obese individuals responses to a 10-wk randomized hypoenergetic diet. Am J Clin Nutr. 2010;91:4729. [PubMed] [Google Scholar]120.
Lffler D, Behrendt S, Creemers JWM, et al. Functional and clinical relevance of novel and known PCSK1 variants for childhood obesity and glucose metabolism. Mol Metab. 2016;6:295305. [PMC free article] [PubMed] [Google Scholar]121.
Huang T, Huang J, Qi Q, et al. PCSK7 genotype modifies effect of a weight-loss diet on 2-year changes of insulin resistance: the POUNDS LOST trial. Diabetes Care. 2015;38:43944. [PMC free article] [PubMed] [Google Scholar]123.
Lin X, Eaton CB, Manson JE, Liu S. The genetics of physical activity. Curr Cardiol Rep. 2017;19:119. [PubMed] [Google Scholar]124.
Lin X, Zhang X, Guo J, et al. Effects of exercise training on cardiorespiratory fitness and biomarkers of cardiometabolic health: a systematic review and meta-analysis of randomized controlled trials. J Am Heart Assoc. 2015;4:e002014. [PMC free article] [PubMed] [Google Scholar]125.
Goryakin Y, Aldea A, Lerouge A, et al. Promoting sport and physical activity in Italy: a cost-effectiveness analysis of seven innovative public health policies. Ann Ig. 2019;31:61425. [PubMed] [Google Scholar]126.
Carlson SA, Fulton JE, Pratt M, Yang Z, Adams EK. Inadequate physical activity and health care expenditures in the United States. Prog Cardiovasc Dis. 2015;57:31523. [PMC free article] [PubMed] [Google Scholar]127.
De Geus EJ, De Moor MH. Genetic and molecular aspects of sport performance. Oxford: Blackwell Publishing; 2011. Genes, exercise, and psychological factors. [Google Scholar]128.
Perusse L, Tremblay A, Leblanc C, Bouchard C. Genetic and environmental influences on level of habitual physical activity and exercise participation. Am J Epidemiol. 1989;129:101222. [PubMed] [Google Scholar]129.
Simonen RL, Perusse L, Rankinen T, Rice T, Rao DC, Bouchard C. Familial aggregation of physical activity levels in the Quebec Family Study. Med Sci Sports Exerc. 2002;34:113742. [PubMed] [Google Scholar]130.
Sallis JF, Patterson TL, Buono MJ, Atkins CJ, Nader PR. Aggregation of physical activity habits in Mexican-American and Anglo families. J Behav Med. 1988;11:3141. [PubMed] [Google Scholar]131.
Choh AC, Demerath EW, Lee M, et al. Genetic analysis of self-reported physical activity and adiposity: the Southwest Ohio Family Study. Public Health Nutr. 2009;12:105260. [PMC free article] [PubMed] [Google Scholar]132.
Butte NF, Cai G, Cole SA, Comuzzie AG. Viva la Familia Study: genetic and environmental contributions to childhood obesity and its comorbidities in the Hispanic population. Am J Clin Nutr. 2006;84:64654. [PubMed] [Google Scholar]133.
Moore LL, Lombardi DA, White MJ, Campbell JL, Oliveria SA. RC. Influence of parents physical activity levels on activity levels of young children. J Pediatr. 1991;118:2159. [PubMed] [Google Scholar]134.
Risch N, Merikangas K. The future of genetic studies of complex human diseases. Science. 1996;273:15167. [PubMed] [Google Scholar]135.
Altmuller J, Palmer LJ, Fischer G, Scherb H, Wjst M. Genome-wide scans of complex human diseases: true linkage is hard to find. Am J Hum Genet. 2001;69:93650. [PMC free article] [PubMed] [Google Scholar]136.
Simonen RL, Rankinen T, Perusse L, et al. Genome-wide linkage scan for physical activity levels in the Quebec Family Study. Med Sci Sports Exerc. 2003;35:13559. [PubMed] [Google Scholar]137.
Cai G, Cole SA, Butte N, et al. A quantitative trait locus on chromosome 18q for physical activity and dietary intake in Hispanic children. Obesity (Silver Spring) 2006;14:1596604. [PubMed] [Google Scholar]138.
De Moor MH, Posthuma D, Hottenga JJ, Willemsen G, Boomsma DI, De Geus EJ. Genome-wide linkage scan for exercise participation in Dutch sibling pairs. Eur J Hum Genet. 2007;15:12529. [PubMed] [Google Scholar]139.
Stefan N, Vozarova B, Del Parigi A, et al. The Gln223Arg polymorphism of the leptin receptor in Pima Indians: influence on energy expenditure, physical activity and lipid metabolism. Int J Obes Relat Metab Disord. 2002;26:162932. [PubMed] [Google Scholar]140.
Richert L, Chevalley T, Manen D, Bonjour JP, Rizzoli R, Ferrari S. Bone mass in prepubertal boys is associated with a Gln223Arg amino acid substitution in the leptin receptor. J Clin Endocrinol Metab. 2007;92:43806. [PubMed] [Google Scholar]141.
De Moor MH, Liu YJ, Boomsma DI, et al. Genome-wide association study of exercise behavior in Dutch and American adults. Med Sci Sports Exerc. 2009;41:188795. [PMC free article] [PubMed] [Google Scholar]142.
Voisin S, Eynon N, Yan X, Bishop DJ. Exercise training and DNA methylation in humans. Acta Physiol (Oxf) 2015;213:3959. [PubMed] [Google Scholar]143.
Krieger JW, Sitren HS, Daniels MJ, Langkamp-Henken B. Effects of variation in protein and carbohydrate intake on body mass and composition during energy restriction: a meta-regression. Am J Clin Nutr. 2006;83:26074. [PubMed] [Google Scholar]144.
Knapik JJ. The importance of physical fitness for injury prevention: part 2. J Spec Oper Med. 2015;15:1125. [PubMed] [Google Scholar]145.
Van Deveire KN, Scranton SK, Kostek MA, et al. Variants of the ankyrin repeat domain 6 gene (ANKRD6) and muscle and physical activity phenotypes among European-derived American adults. J Strength Cond Res. 2012;26:17408. [PMC free article] [PubMed] [Google Scholar]146.
Bruneau M Jr, Walsh S, Selinsky E, et al. A genetic variant in IL-15Ra correlates with physical activity among European-American adults. Mol Genet Genomic Med. 2018;6:4018. [PMC free article] [PubMed] [Google Scholar]147.
Gielen M, Westerterp-Plantenga MS, Bouwman FG, et al. Heritability and genetic etiology of habitual physical activity: a twin study with objective measures. Genes Nutr. 2014;9:415. [PMC free article] [PubMed] [Google Scholar]148.
Akhmetov II, Astranenkova IV, Rogozkin VA. Mol Biol (Mosk) 2007;41:8527. [PubMed] [Google Scholar]149.
Stefan N, Vozarova B, Del Parigi A, et al. The Gln223Arg polymorphism of the leptin receptor in Pima Indians: influence on energy expenditure, physical activity and lipid metabolism. Int J Obes Relat Metab Disord. 2002;26:162932. [PubMed] [Google Scholar]150.
De Moor MH, Posthuma D, Hottenga JJ, Willemsen G, Boomsma DI, De Geus EJ. Genome-wide linkage scan for exercise participation in Dutch sibling pairs. Eur J Hum Genet. 2007;15:12529. [PubMed] [Google Scholar]151.
Lorentzon M, Lorentzon R, Lerner UH, Nordstrom P. Calcium sensing receptor gene polymorphism, circulating calcium concentrations and bone mineral density in healthy adolescent girls. Eur J Endocrinol. 2001;144:25761. [PubMed] [Google Scholar]152.
Winnicki M, Accurso V, Hoffmann M, et al. Physical activity and angiotensin-converting enzyme gene polymorphism in mild hypertensives. Am J Med Genet A. 2004;125A:3844. [PubMed] [Google Scholar]153.
Loos RJ, Lindgren CM, Li S, et al. Common variants near MC4R are associated with fat mass, weight and risk of obesity. Nat Genet. 2008;40:76875. [PMC free article] [PubMed] [Google Scholar]154.
Kilpelinen TO, Qi L, Brage S, et al. Physical activity attenuates the influence of FTO variants on obesity risk: a meta-analysis of 218,166 adults and 19,268 children. PLoS Med. 2011;8:e1001116. [PMC free article] [PubMed] [Google Scholar]155.
Rankinen T, Rice T, Teran-Garcia M, Rao DC, Bouchard C. FTO genotype is associated with exercise training-induced changes in body composition. Obesity. 2010;18:3226. [PMC free article] [PubMed] [Google Scholar]156.
Cassidy S, Chau JY, Catt M, et al. Cross-sectional study of diet, physical activity, television viewing and sleep duration in 233110 adults from the UK Biobank; the behavioural phenotype of cardiovascular disease and type 2 diabetes. BMJ Open. 2016;6:e010038. [PMC free article] [PubMed] [Google Scholar]157.
Franks PW, Jablonski KA, Delahanty LM, et al. Assessing gene-treatment interactions at the FTO and INSIG2 loci on obesity-related traits in the Diabetes Prevention Program. Diabetologia. 2008;51:221423. [PMC free article] [PubMed] [Google Scholar]158.
Liaw YC, Liaw YP, Lan TH. Physical activity might reduce the adverse impacts of the FTO gene variant rs3751812 on the body mass index of adults in Taiwan. Genes (Basel) 2019;10:354. [PMC free article] [PubMed] [Google Scholar]159.
Bruneau M Jr, Angelopoulos TJ, Gordon P, et al. The angiotensin-converting enzyme insertion/deletion polymorphism rs4340 associates with habitual physical activity among European American adults. Mol Genet Genomic Med. 2017;5:52430. [PMC free article] [PubMed] [Google Scholar]160.
Khoury MJ, Gwinn M, Bowen MS, Dotson WD. Beyond base pairs to bedside: a population perspective on how genomics can improve health. Am J Public Health. 2012;102:347. [PMC free article] [PubMed] [Google Scholar]161.
San-Cristobal R, Milagro FI, Martinez JA. Future challenges and present ethical considerations in the use of personalized nutrition based on genetic advice. J Acad Nutr Diet. 2013;113:144754. [PubMed] [Google Scholar]162.
Bergmann MM, Gorman U, Mathers JC. Bioethical considerations for human nutrigenomics. Annu Rev Nutr. 2008;28:44767. [PubMed] [Google Scholar]163.
Arab L. Individualized nutritional recommendations: do we have the measurements needed to assess risk and make dietary recommendations? Proc Nutr Soc. 2004;63:16772. [PubMed] [Google Scholar]166.
Human Genetic Commission (UK HGC) A common framework of principles for direct-to-consumer genetic testing services. London, Human Genome Commission. 2010 [Google Scholar]167.
The German Human Genetic Examination Act (Gesetz ber genetische Untersuchungen bei Menschen Gendiagnostikgesetz-GenDG) 2009 [Google Scholar]168.
Superior Health Council: Publication of the Superior Health Council No. 8714. Brussels, Superior HealthCouncil, 2012. [Google Scholar]169.
Borry P, van Hellemondt RE, Sprumont D, et al. Legislation on direct-to consumer genetic testing in seven European countries. Eur J Hum Genet. 2012;20:71521. [PMC free article] [PubMed] [Google Scholar]171.
Berry S, Valdes A, Davies R, et al. Predicting personal metabolic responses to food using multi-omics machine learning in over 1000 twins and singletons from the UK and US: The PREDICT I Study (OR31-01-19) Curr Dev Nutr. 2019:3. [Google Scholar]172.
Stanton MV, Robinson JL, Kirkpatrick SM, et al. DIETFITS study (diet intervention examining the factors interacting with treatment success) - Study design and methods. Contemp Clin Trials. 2017;53:15161. [PMC free article] [PubMed] [Google Scholar]